GROWTH PATTERN ANALYSIS OF SPRING SOWN CAMELINA [CAMELINA SATIVA L. (CRANTZ.)] DUE TO VARIATION OF SOWING TIME
M. Göre1*, O. Kurt1and H. Uysal2
1Ondokuz Mayis University, Faculty of Agriculture, Department of Field Crops, Samsun-Turkey.
2Adnan Menderes University, Faculty of Agriculture, Department of Biotechnology, Aydın-Turkey.
*Corresponding Author’s e-mail: merve.gore@ege.edu.tr
ABSTRACT
This research was conducted to determine the change of main growth parameters and growth pattern of camelina depending on the sowing time and genotype. The research was carried out during 2017 and 2018 and the treatments (1, 11, 21 and 31 May & PI-650142 and PI-304269 genotypes) were arranged in split-plot design and 3 replications. It was determined that NAR, LAI, RGR and DM were affected by the sowing times. In addition, NAR, LAI and RGR were significantly affected by genotypes at all growth stages, whereas DM was affected only in the pre-flowering stage. Depending on the sowing times and genotype, NAR was found 0.014-0.016, 1.65-1.96 and 4.10-15.51 mg mm-2 day-1 in pre-flowering, flowering and post-flowering periods, respectively, while LAI was found 0.22-0.29, 0.23-0.33 and 0.10-0.23 mm2 mm-2, respectively. In pre-flowering, flowering, post-flowering and harvest period RGR was found 2.3-2.6, 3.3-4.4, 2.2-3.3 and 0.6-0.7 mg g-1 day-1 g-1 day-1, respectively, while DM was 0.233-0.283, 0.410-0.553, 0.646- 0.944 and 0.939-1.003 g per plant, respectively. Also, depending on the sowing time, the seed yield was determined as 190.85-810.07 kg ha-1 for the PI-650142 genotype and 190.94-360.65 kg ha-1 for the PI-304269 genotype. Consequently, it can be suggested that PI-650142 genotype can be used for general sowing once date is adjusted to 1 May in conditions of Samsun. This could be contributed to optimum temperature and day length during the flowering, seed formation and filling stage.
Keywords: camelina; sowing time; growth pattern; NAR; LAI; RGR; DM; Seed yield
https://doi.org/10.36899/JAPS.2022.1.0415
Published online June 14, 2021
INTRODUCTION
Growth is defined as the numerical increase of plant parts or the irreversible change of dry matter in the size of the plant. In other words, growth can also be referred to as the net increase in the dry matter of each plants’ leaf area per unit time (Charles-Edwars et al., 1986). Determination of some physiological parameters such as net assimilation rate (NAR), leaf area index (LAI), relative growth rate (RGR) and total dry matter (DM) which are considered as determinants of the growth and development of plants, plays a major role in determining growth ultimately the crop productivity (Koutroubas et al., 2012).
Leaves receive light from the sun and use it in photosynthesis (Ackerly et al., 2002). It affects various physiological processes directly or indirectly, such as photosynthesis, respiration, transcription, which are vital in leaf area, biosphere and atmosphere relationships (Guo and Sun, 2001). During vegetative growth, the leaf area index (LAI) increases as the number of leaves increases but decreases again with leaf aging towards the time of maturing (Ackerly et al., 2002). Therefore, leaf area index is one of the important parameters used in plant analysis due to the role of leaf characteristics in carbon assimilation, water relations and energy balance (Charles-Edwars et al., 1986). Measurement of leaf area is also important in plant nutrition, plant competition, plant soil-water relations, light reflection and heat transfer in heating and cooling processes (Chen, 2009).
Relative Growth Rate (RGR) is a key variable of critical importance in plant physiology and is an important parameter for determining the response of plants to different environments and different treatments (Grime, 2001). Thus, it has been stated that RGR is an effective factor in measuring the amount of dry matter accumulation (Beadle, 1993).
The proportional expression of plant growth occurs in a geometrically folded manner depending on time, temperature and light (Hadley et al., 1983). Growth analyses include useful and complex analyses that determine the interaction between growth and plant ecology. Plant growth analysis is necessary to explain the differences in plant growth between the species that grow under the same or different environmental conditions. It is not only the effect of genetic structure, but also the effects of environmental variance on plant growth, seed yield, and quality parameters are crucial factors (Kurt, 2015). Any changes that may occur in the ecology of the plant during plant growth can directly or indirectly affect the physiology of the plant (Hunt, 1990).
Environmental parameters, especially temperature and photoperiod, play an effective role in the realization of the physiological process in the plant (Hatfield and Prueger, 2015). Appropriate temperature and light contribute to the occurrence of physiological events such as photosynthesis, respiration and transpiration by ensuring the regular operation of enzymes and hormones related to growth and development in the plant which effect the crop yield at the end. Consequently, the ecological and climatic factors of production areas are important in the decision-making process of determining plant, species and variety (Uzun and Demir, 1996).
Camelina sativa (L.) Crantz belongs to the family of Brassicaceae and only economically important species in this genus (Davis, 1965; Gore and Kurt, 2015). Camelina is the raw material for many products in biodiesel production, oil industry and machine (Sawyer, 2008; Liu et al., 2013). In addition, it is accepted as a source of cholesterol-lowering vegetable oil in human nutrition due to the high content of Omega-3 (linolenic acid) fatty acid (Karvonen et al., 2002). Camelina seeds contain 35-40% oil; about 90% of this is unsaturated fatty acids, and about 10% of this is saturated fatty acids (Zubr, 1997). In addition to this, it is a plant that can adapt to marginal soils, does not require much input (fertilizers, irrigation, insecticide etc.), can be evaluated in summer and winter grown, and has a short vegetation period (Kurt and Seyis, 2008; Ahmed et al., 2019).
As with the other cultivated plants, the agronomic characteristics of camelina are influenced by the growing season, location and year, and environmental conditions that vary between these factors. Also, these features are significantly affected by cultural practices such as sowing time, fertilization, irrigation and tillage management (Kirkhus et al., 2013; Neupane et al., 2019). A change of sowing time necessarily involves changes in climatic parameters such as temperature, light and moisture that affect the plant in different growth and development periods, thereby affecting the growth pattern of the plant (Koca and Turgut, 2012).
Phenological growth periods of camelina plant were defined according to extended BBCH scale as germination, cotyledon development, the formation of side shoot, elongation of the main stem, development of vegetative parts, flowering, development of capsule, maturing, and senescence (Martinelli and Galasso, 2011).
Although many studies have been done on different aspects of camelina, there is almost no research in which growth parameters are measured, especially during developmental periods. As the final goal; to eliminate the negative effects of environmental factors on the plant, it is to determine the development period of the plant to which it is most sensitive and to make recommendations to take the necessary measures. Therefore, the present research carried out to know the effect of sowing time on main growth parameters and seed yield of camelina.
MATERIALS AND METHODS
Plant Material: PI-650142 (Denmark) and PI-304269 (Sweden) camelina genotypes provided from USDA (United States Department of Agriculture) were used as plant materials. As a result of the preliminary researches, these genotypes were used in this research because of the best adaptation to the research field in terms of yield and yield elements.
Experimentation: Field experiments were conducted at Samsun-Turkey (41°37.49'N, 35°36'30"E) in spring vegetation period in 2017 and 2018. When the climate data of the research period is examined, it is observed that there are differences in the two vegetation periods at various times. Monthly average temperature, day length and humidity values are higher than the average of the experiment in the 2nd year (2018), 1st year (2017) and long years. Monthly average precipitation values are lower than the average of long years in both years of the experiment. In the first year of the experiment, there was no rainfall in July (Figure 1). The soil structure of the experiment area is clay loam, its organic substance (2.83%) and nitrogen content are moderate, K2O concentration is high (exchangeable K 7.59 kg ha-1), P2O5 concentration is medium (available P 0.66 kg ha-1), lime content 1.73% and soil pH is 7.71.
Fig. 1. The average monthly temperature, day length, rainfall and relative humidity recorded during the camelina growing season in 2017 and 2018 (Anonymous, 2019).
The plots for the experiments were arranged in a split-plot design with three replicates, in which varieties were set up as the main plot, whereas sowing times as the sub-plot. The dates for sowing were arranged at May 1, 11, 21 and 31 during the 2017 and 2018 summer seasons. Also, four different sampling periods (pre-flowering, flowering, post-flowering and harvesting) were used in both years. The experimental area was empty in the previous year, the field was plowed twice according to the cultivation of camelina and suitable sowing intervals were opened. Plot size was 3x1 m with a spacing of 20 cm row distance and 5 cm plant distance in each row. Camelina genotypes were sown using the sprinkle sowing method by hand. In both years of the experiment period; 40 kg ha-1 of ammonium nitrate fertilizer was applied before flowering. Weed control was carried out twice, before the pre-flowering and during the full-flowering stage. Field capacity irrigation was carried out on 1 July in the first year and on 12 June and 11 July in the second year. The plants harvested by hand when 90% of the capsules had matured in both years.
Parameter calculation and statistical analysis: Data were calculated according to the formulae proposed by Taiz et al., (2014). The formulae used in calculations are given below.
NAR= (1/LA) (dW/dt); LAI= LA/ Ground Area; RGR= (1/W) (dW/dt)
Among the abbreviations in the formulas, LA: Leaf Area, dW: Dry weight change between sampling periods and dt: it refers to the time between two quantitative analyses.
10 plants' leaves samples taken randomly from each plot in each sampling period were made according to O’Neal et al., (2002) using "Imaje J Image Analysis Program".
The data were statistically analysed according to using SPSS 17.0 statistical software. Homogeneity test was done for each parameter and the means of treatments were compared by DUNCAN's Multiple Range Test at 5% and 1% probability level.
RESULTS AND DISCUSSION
Net assimilation rate (NAR): The effect of different sowing times, genotypes, years, genotype × year (G×Y) and year × sowing time (Y×S) was very significant (P< 0.01) on net assimilation rate in the pre-flowering stage. While sowing time effects were significant (P< 0.05); year, genotype, Y×S, G×Y and genotype × sowing time (G×S) was very significant (P< 0.01) in the flowering stage. The variation of all traits was significant (P< 0.01 and P< 0.05) in the post-flowering period (Table 1). According to pre-flowering, flowering and post-flowering period, NAR was 0.008, 2.045 and 11.193 mg mg-2 day-1 in the first year, respectively, while the second year is 0.021, 1.549 and 7.757 mg mg-2 day-1. The difference between years was mainly due to the difference in precipitation and day length (Fig. 1). Compared with the first year, in the second year, the day length and drought period were especially long after the flowering period.
The NAR increased in all sowing times and during the growth period in both genotypes, and this increase continued rapidly from the flowering period (Fig. 2). In the pre-flowering, flowering and post-flowering periods, NAR were 0.014, 1.653 and 8.482 mg mg-2 day-1 in the PI-650142 genotype, while it was 0.015, 1.941 and 10.467 mg mg-2 day-1 in the PI-304269 genotype, respectively. This progress in vegetative growth causes the acceleration of physiological stages such as photosynthesis and transpiration. Therefore, the net assimilation rate increased rapidly from flowering stages.
Depending on the genotypes and sowing times, the net assimilation rate changed between 0.014-0.017 mg mg-2 day-1 in the pre-flowering period, 1.496-2.03 mg mg-2 day-1 in the flowering period and 3.58-17.98 mg mg-2 day-1 in the post-flowering period. In both genotypes, the highest values (17.98 mg mg-2 day-1) were obtained from the first sowing time in all growth stages. The PI-304269 genotype has reached the highest value of NAR in all growth stages. The NAR increase in both genotypes decreased from the flowering to the post-flowering period as the sowing time was delayed. The lowest NAR was obtained for the last sowing time. As a matter of fact, it has been reported that lower NAR is obtained in the case of late sowing since the vegetative period is completed in a shorter time and the generative period is switched (Board, 2000).
Table 1. Variance analysis table and F values of the NAR and LAI for the growth periods.
Variation
Sources
|
df
|
NAR
|
LAI
|
General
|
47
|
Pre-Flowering
|
Flowering
|
Post-Flowering
|
Pre-Flowering
|
Flowering
|
Post-Flowering
|
Year (Y)
|
1
|
2853.02 **
|
118.75 **
|
1234.67 **
|
11.42 **
|
21.67 **
|
31.78 **
|
Block
|
4
|
0.66 NS
|
0.54 NS
|
0.63 NS
|
0.72 NS
|
2.70 NS
|
0.80 NS
|
Error1
|
4
|
|
|
|
|
|
|
Genotype (G)
|
1
|
17.74 **
|
90.73 **
|
505.41 **
|
40.43 **
|
158.22 **
|
142.51 **
|
G×Y
|
1
|
13.04 **
|
74.81 **
|
56.06 **
|
115.72 **
|
23.55 **
|
204.71 **
|
Error2
|
12
|
|
|
|
|
|
|
Sowing Time (S)
|
3
|
23.58 **
|
8.38 *
|
2684.89 **
|
42.10 **
|
213.73 **
|
269.94 **
|
Y×S
|
3
|
20.42 **
|
29.32 **
|
2519.77 **
|
198.93 **
|
802.85 **
|
333.98 **
|
G×S
|
3
|
3.42 NS
|
21.83 **
|
92.29 **
|
198.72 **
|
43.38 **
|
84.85 **
|
G×S×Y
|
3
|
2.32 NS
|
7.14 NS
|
7.07 *
|
46.78 **
|
272.13 **
|
72.55 **
|
Error3
|
12
|
|
|
|
|
|
|
CV (%)
|
|
4.71
|
6.30
|
5.05
|
6.27
|
4.67
|
5.06
|
Note: P< 0.05(*), P< 0.01(**), Non-significant (NS)
The NAR effects the amount of dry matter produced by leaves. In late sowing times, the increase in temperature and shortening of the vegetation period led to a decrease in the NAR and leaf area (Martinelli and Galasso, 2011; Fujita et al., 2014). In the post-flowering period, the photosynthetic rate decreases with the aging of leaves and the accumulation of dry matter in the leaf, thus the NAR decreases rapidly until harvest. In addition, the plants' stomas close during the drought that occurs with high temperatures. NAR decreases in plants exposed to high temperatures at the end of the vegetative period (Russo et al., 2010).
It has been reported that NAR varies between 4.00-5.24 mg mm-2 d-1 in 35 days after sowing under water stress conditions (Waraich et al., 2017a). In a study conducted to optimize the sowing time of two different camelina genotypes under drought stress in terms of growth potential, it was determined that the growth parameters were affected by the sowing time difference. While maximum values were obtained from early sowing, minimum values were determined from late sowing and it was determined that the NAR varies between 3.5-5.40 mg mm-2 d-1 (Waraich et al., 2017b).
Fig. 2. Effects of different sowing times on NAR of two camelina varieties at different growth stages.
Leaf area index (LAI): The effect of different sowing times, genotypes, years and all interactions were highly significant (P< 0.01) on LAI at all growth stages (Table 1). Depending on the genotypes and sowing times, the LAI changed between 0.205-0.362 mm2 mm-2 in the pre-flowering period; 0.255-0.360 mm2 mm-2 in the flowering period and 0.079-0.244 mm2 mm-2 in the post-flowering period. When the data of the two years are evaluated together; the highest LAI value was obtained at the 3rd sowing time with 0.297 and 0.239 mm2 mm-2 values before and after flowering, respectively, while the highest value at the flowering time was obtained with 0.333 mm2 mm-2 at the 1st sowing time. The LAI increased in all sowing times in both genotypes until the flowering period and then started to decrease (Fig. 3). This situation was due to the cessation of leaf formation and the yellowing and shedding of existing leaves after the flowering period.
Determining the LAI in plants is of great importance in terms of evaluating growth and physiological analysis. Day length is an important factor in increasing LAI (Charles-Edwars et al., 1986). Early spring sowing is effective in taking advantage of day length, and LAI is higher, especially in later stages of the vegetative development period. As a matter of fact, in this study, it was determined that day length and LAI both increased until the flowering period and decreased depending on the day length in the next period (Fig.1; 3).
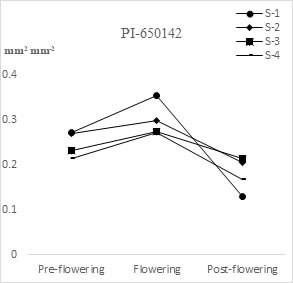 
Fig. 3. Effects of different sowing times on LAI of two camelina varieties at different growth stages.
The LAI affects various physiological processes and energy balance directly or indirectly, such as photosynthesis, respiration, transcription, which are vital in the biosphere and atmosphere relationships (Guo and Sun, 2001; Ackerly et al., 2002; Chen, 2009). The optimum LAI in the flowering period is expressed as the main determinants of yield (Tagliapietra et al., 2018). LAI decreased due to shading of the lower leaves, mobilization of nutrients from leaves to capsule and leaf senescence until harvesting time reached (Brunel-Muguet et al., 2013; Waraich et al., 2017b). As a matter of fact, in this research, it was determined that RGR decreased due to the decrease in LAI after flowering (Fig 3; 4).
It has been shown that the LAI was affected by the sowing time in camelina (Neupane et al., 2019). Also, several researchers have reported effects of sowing times on growth parameters of camelina (Sintim et al., 2016; Ahmed et al., 2019). Sowing delay results in shortening of the vegetation period and reduced yield due to high temperatures during the seed filling period. Since the LAI is a parameter that affects growth and hence yields, early sowing of spring is expected to have higher performance in terms of these parameters. In this research, the maximum increase rate between pre-flowering and flowering period was obtained from the first sowing times in both genotypes (30.8% for PI-650142; 46.9% for PI-304269). Similarly, the maximum decrease between flowering and post-flowering period was obtained from the first sowing time in both genotypes (60.0% for PI-650142; 78.0% for PI-304269).
Relative growth rate (RGR): The effect of different sowing times was significant (P< 0.01 and P< 0.05) on RGR in all growth periods except harvesting period. The effect of genotypes was only highly significant (P< 0.01) in the pre-flowering period. Year × Sowing times interaction was significant (P< 0.01) in all periods. The importance of degrees of interactions change according to the growth periods (Table 2). PI-650142 genotype showed the highest result in the pre-flowering period with 2.6 mg g-1 day-1. Depending on the genotypes and sowing times, the RGR changed between 2.2-2.6 mg g-1 day-1 in the pre-flowering period, 3.3-4.4 mg g-1 day-1 in the flowering period, 2.2-3.4 mg g-1 day-1 in the post-flowering period and 0.6-0.8 mg g-1 day-1 in the harvest period.
Table 2. Variance analysis table and F values of the RGR and total dry matter for the growth periods.
Variation
Sources
|
df
|
RGR
|
DM
|
General
|
47
|
Pre-flowering
|
Flowering
|
Post-flowering
|
Harvest
|
Pre-flowering
|
Flowering
|
Post-flowering
|
Harvest
|
Year (Y)
|
1
|
60.70 **
|
0.03 NS
|
27.28 **
|
1.46 NS
|
5.27 *
|
6.24 *
|
878.98 **
|
6082.18 **
|
Block
|
4
|
2.62 NS
|
1.00 NS
|
0.35 NS
|
1.59 NS
|
2.14 NS
|
0.50 NS
|
1.10 NS
|
3.78 NS
|
Error1
|
4
|
|
|
|
|
|
|
|
|
Genotype (G)
|
1
|
8.92 **
|
1.09 NS
|
1.40 NS
|
0.37 NS
|
12.01 **
|
7.62 *
|
315.62 **
|
583.31 **
|
G×Y
|
1
|
0.18 NS
|
20.11 **
|
16.45 **
|
0.50 NS
|
31.19 **
|
134.90 **
|
644.63 **
|
1.99 NS
|
Error2
|
12
|
|
|
|
|
|
|
|
|
Sowing Time (S)
|
3
|
22.43 **
|
5.59 *
|
83.28 **
|
0.88 NS
|
16.69 **
|
50.37 **
|
348.88 **
|
701.20 **
|
Y×S
|
3
|
25.12 **
|
24.02 **
|
140.24 **
|
26.89 **
|
200.94 **
|
234.63 **
|
420.52 **
|
1269.23 **
|
G×S
|
3
|
1.12 NS
|
5.87 *
|
5.07 *
|
0.80 NS
|
218.71 **
|
140.17 **
|
41.24 **
|
156.98 **
|
G×S×Y
|
3
|
1.39 NS
|
7.74 *
|
0.33 NS
|
7.29 *
|
53.65 **
|
118.81 **
|
7.30 *
|
297.43 **
|
Error3
|
12
|
|
|
|
|
|
|
|
|
CV (%)
|
|
3.27
|
12.67
|
8.23
|
19.16
|
5.89
|
4.02
|
3.20
|
2.72
|
Note: P< 0.05(*). P< 0.01(**). Non-significant (NS)
The RGR was highest at last sowing time in all growth stages. While the highest RGR value was obtained at the 4th sowing time with 4.4, 3.3 and 0.7 mg g-1 day-1 in the flowering, post-flowering and harvest periods, respectively, while the pre-flowering period was obtained with 2.6 mg g-1 day-1 at the 3rd sowing time. The rapid growth period in both genotypes continued until the flowering period and then began to decline to the harvest (Fig. 4).
Maximum RGR increase in both genotypes (PI-650142 and PI-304269) with a value of 91.3% and 100.0% respectively were obtained between pre-flowering and flowering period on the last sowing time. In contrast, the maximum RGR rate decline between flowering and post-flowering period was obtained from the time of first sowing in both genotypes with values 33.3% for PI-650142 and 47.6% for PI-304269. The highest RGR decrease value between the post-flowering and the harvest period was obtained from the last sowing time in PI-650142 genotype with a value of 79.4% and from second sowing time in PI-304269 genotype with a value of 82.3%.
Fig. 4. Effects of different sowing times on RGR of two camelina varieties at different growth stages.
RGR is an important parameter to determine the plants' reactions to different environments and different applications. Indeed, it has been stated that the RGR is an effective factor in measuring the amount of dry matter and the primary determinant of RGR is NAR (Veneklaas and Poorter, 1998). Srivastava and Singh (1980) reported that RGR and NAR directly affect economic efficiency. The RGR value of a plant reflects its vigour and is considered a good index of its exposure to stresses of all sorts (Lutts et al., 2004). In this research, the camelina growing at varying sowing times revealed a decrease in RGR with increasing temperature at critical growth stages as the flowering period. In the last phase of the growth period, RGR has decreased to zero due to the increase in dead and woody tissues compared to living and active tissues. Similar results were also obtained from Brassica rapa, which is from the same family as camelina (Yasari and Patwardhan, 2006). Poorter and Remkes (1990) reported that not find a relationship between NAR and RGR in their research on many plant species in opposite environmental conditions. According to the findings obtained in this research, it was determined that there was a reverse relationship between NAR and RGR, and this relationship emerged more clearly especially from the flowering period.
In researches conducted to determine the effects of different day lengths on RGR and growth components; although day length has been reported to have a significant effect on RGR (Shipley, 2002). On the other hand, other researchers have been reported to have no effect (Poorter and van der Werf, 1998). Relationships between RGR and its components may vary according to genotype and environmental factors. In the first year of this research, a significant decrease is observed in the day length parameter after the flowering period. The reason for the rapid decrease after the flowering period may be due to differences in the day length (Fig. 1; 4).
Total Dry Matter (DM): The effect of different sowing times, genotypes, years and all interactions except for G×Y interaction at harvest and trio-interaction at the flowering period was significant (P< 0.01 and P< 0.05) on a DM (Table 2). Except after flowering, the highest DM amount was obtained at the 1st sowing time with 0.283, 0.553 and 1.003 g in all other periods including pre-flowering, flowering and harvest periods, respectively, while it was obtained at the 2nd sowing time with 0.944 g after flowering.
DM amount continued to increase in all development periods and harvest time reached its maximum. Considering all development periods; in the PI-650142 genotype compared to other sowing times, the DM amount at the first sowing time increased significantly until the harvest time, while in the PI-304269, the DM amount at the second sowing time until the end of the flowering period increased significantly compared to other sowing times (Fig. 5). The rapid growth period in both genotypes continued to until the flowering period, and high DM in the plants was owing to the increase in the weight of dry leaves. This appears as a result of the increase in the number of leaves and the leaf area index. After the flowering period, DM accumulation continued to a slow growth period and DM weight increased slightly into the harvest period.
Depending on the genotypes and sowing time, the DM changed between 0.188-0.366 g in the pre-flowering period, 0.359-0.594 g in the flowering period, 0.631-1.005 g in the post-flowering period and 0.765-1.242 g in the harvest period. The PI-650142 genotype has a higher DM than the PI-304269 genotype in all growth periods except the flowering period.
DM build-up mainly effects by the amount of radiation captured by the product. As the respiration increases in the later stages of growth, high temperature affects DM (Hussain, 1994). As maturation progresses, DM accumulation increases (Peiretti and Meineri, 2007). A similar situation was found in this research. The decrease in the rate of increase of the DM after the flowering period may be the result of total ageing of the leaves, the death of vegetative parts and severe droughts during the maturity period. The DM, which has increased after the flowering period, makes important contributions to the harvest index and increases the seed yield (Baydar and Kara, 2010). Because of this, the life cycle of camelina grown during summer vegetation is short, it tends to complete the vegetative development and move to the generative period due to the high temperature and day length in this research. Consequently, DM in summer plants accumulates faster during the vegetative growth phase. The differences between the years of the DM production and the accumulation pattern in sowing times may be the result of climatic changes between years. The weather was relatively warm and moist during the second year of this research, which would have shortened the vegetative growth phase (Fig. 1).
Fig. 5. Effects of different sowing times on DM of two camelina varieties at different growth stages.
Seed yield: The effect of different sowing times, genotypes, years and all interactions at the harvest was significant (P< 0.01 and P< 0.05) on seed yield (Table 3). The average seed yield of PI-650142 genotype was 530.0 kg ha-1 while the seed yield of PI-304269 genotype was 300.5 kg ha-1. When the research result is evaluated on the sowing time; the highest seed yield was obtained from the 1st sowing time (580.9 kg ha-1), while the lowest seed yield was obtained from the 4th sowing time (190.9 kg ha-1). When G×EZ interaction in seed yield is evaluated, the highest seed yield was obtained from the 1st sowing time (810.1 kg ha-1) of the PI-650142 genotype. It was determined that the lowest seed yield was obtained from the 4th sowing time (190.9 and 190.8 kg ha-1) of both genotypes (Fig. 6).
According to the result of the previous research, seed yield was reported as 340.4-790.8 kg ha-1 in spring sown camelina (Kınay et al., 2019). The reported result is in line with the results obtained in our research. However, seed yield was reported by another researcher as 1900.0 kg ha-1 (Kurasiak-Popowska et al., 2018). Genetic structure of genotypes, environmental conditions and cultural practices are the main criteria affecting seed yield. Although cultural practices have a serious impact on seed yield, the aim of this study is to determine the effect of sowing time on the basic growth parameters of camelina. Therefore, while evaluating this research, the effects of cultural practices were not emphasized. It has been reported by many researchers that dry matter is one of the important parameters affecting seed yield and there is a strong relationship between dry matter and seed yield (Hussain, 1994; Hatfield and Prueger, 2015). The seed yield resulting from the growth and development process is the most important character in terms of agriculture. Determining the changes in growth parameters also plays a key role in detecting the appropriate genotypes for seed yield and suitable sowing times.

Fig. 6. Effects of different sowing times on seed yield of two camelina varieties at harvest.
Table 3. Variance analysis table and F values of the seed yield.
Variation Sources
|
df
|
F Value
|
General
|
47
|
|
Year (Y)
|
1
|
14.08 **
|
Block
|
4
|
1.04
|
Error1
|
4
|
|
Genotype (G)
|
1
|
10994.43 **
|
G×Y
|
1
|
4.73 *
|
Error2
|
12
|
|
Sowing Time (S)
|
3
|
13260.04 **
|
Y×S
|
3
|
11.43 **
|
G×S
|
3
|
7592.63 **
|
G×S×Y
|
3
|
22.09 **
|
Error3
|
12
|
|
CV (%)
|
|
1.09
|
CONCLUSION
Growth parameters such as NAR, LAI, RGR and DM and also seed yield are emphasized to determine the plant growth pattern. Determination of the growth pattern is important in terms of revealing the period in which the plant is more sensitive or tolerant throughout its life cycle. These periods can be used as a guide in the cultural processes to be performed during the growth and development period of the plants. It can be concluded that early sowing is more advantageous. However, in case of delay in cultivation for any reason, it is more appropriate to use genotypes that can tolerate the negative effect of the delay more as plant material.
Acknowledgments: The authors would like to thank Charles E. Fiero for editing this article.
Disclosure statement: No potential conflict of interest was reported by the author(s).
Funding: Supported by the Ondokuz Mayis University, Turkey, Project No. PYO.ZRT.1904.17.044
REFERENCES
- Ackerly D.D., C.A. Knight, S.B. Weiss, K. Barto and K.P. Starmer (2002). Leaf size, specific leaf area and microhabitat distribution of chaparral woody plants: contrasting patterns in species level and community level analyses. Oecologia, 130: 449-457.
- Ahmed Z., E.A. Waraich, Z. Qi, D. Dongwei-Gui, M. Shreef, H. Hassan-Iqbal and R.N. Shabbir (2019). Physio-biochemical and yield responses of two contrasting Camelina sativa breeding lines under drought stress. Int. J.Agri. Bio. 22: 1187‒1196.
- (2019). The Turkish State Meteorological Service Samsun Regional Directorship, 2017, 2018 and long term. [accessed 2019 Nov 02]. https://www.mgm.gov.tr/
- Baydar H. and N. Kara (2010). Dry matter accumulation in vegetative and generative parts during growth and development periods of safflower (Carthamus tinctorius). J. Nat. App. Sci. 14(2): 148-155.
- Beadle C.L. (1993). Photosynthesis and Production in a Changing Environment: In: Hall D.O., Scurlock J.M.O, Bolharnordenkampfh R., Leegood R.C., Long S.P. (eds.): A Field and Laboratory Manual. Chapman and Hall, London (UK), 36-46 p.
- Board J. (2000). Light interception efficiency and light quality affect yield compensation of soybean at low plant populations. Crop Sci. 40(5): 1285-1294.
- Brunel-Muguet S., P. Beauclair., M. Bataillé, J.C. Avice, J. Trouverie, P. Etienne and A. Ourry. (2013). Light restriction delays leaf senescence in winter oilseed rape (Brassica napus). J. Plant. Growth. Reg. 32: 506–518.
- Charles-Edwards D.A, D. Doley and G.M. Rimmington (1986). Modelling plant growth and development. Sydney Academic Press, Sydney (Australia), 235 p.
- Chen A. (2009). Measurement of plant leaf parameters based on image processing. Journal of Northeast Forestry University, 37: 46-47.
- Davis P.H. (1965). Flora of Turkey and East Islands, Vol:1. University of Edinburg, London (UK), 680 p.
- Fujita K., S. Fujita, T. Fujita, S. Konish, J. Vollmann, P.K. Mohapatra, M. Hosokawa, M. Kuwahara, H. Saneoka and T. Tadano (2014). Source-sink manipulation of Camelina sativa related to grain yield under stressful environment of Hokkaido, Japan. Soil Sci. Plant. Nutri. 60: 156–161.
- Grime J.P. (2001). Plant Strategies, Vegetation Processes, and Ecosystem Properties. John Wiley and Sons, New Jersey (USA), 456 p.
- Gore, M. and O. Kurt (2015). Research to establish effects of explant sources and plant growth regulators on camelina (Camelina sativa Crantz) tiller and plant induction. An. J. Agri. Sci. 30(3): 268-274.
- Guo D.P., Y.Z. Sun (2001). Estimation of leaf area of stem lettuce (Lactuca sativa varangustana) from linear measurements. Indian J. Agri. Sci.71:483-486.
- Hadley P., E.H. Roberts, R.J. Summerfıeld and F.R. Minchin (1983). A quantitative model of reproductive development in cowpea [Vigna unguiculata(L.) Walp.] in relation to photoperiod and temperature, and implications for screening germplasm. Annals. Bot. 51(4): 531–543.
- Hatfield J.L. and J.H. Prueger (2015). Temperature extremes: Effect on plant growth and development. Weather. Climate. Ext., 10: 4‑10.
- Hunt R. (1990). Absolute growth rates: In: Hunt R. (ed.): Basic growth analysis. Springer, Dordrecht (the Netherlands), 17–24 p.
- Hussain A. (1994). Crops and Their Relation to Environment: In: Bashir E. (ed.): Crop Production. National Book Foundation, Islamabad (Pakistan), 27-46 p.
- Karvonen H.M., A. Aro, N.S. Tapola I. Salminen, M.I.J. Uusitupa and E.S. Sarkkinen (2002). Effect of linolenic acid–rich Camelina sativa oil on serum fatty acid composition and serum lipids in Hyper cholesterolemic subjects. Metabolism. 51(10): 1253-1260.
- Kınay, A, G. Yılmaz, S. Ayısıgı, S. Dokulen, (2019). Yield and quality parameters of winter and summer-sown different camelina (Camelina sativa) genotypes. Turk. J. Field Crops, 24(2): 164-169.
- Kirkhus B., A.R. Lundon, J.H. Haugen, G. Vogt, G.I.A. Borge and B.I.F. Henriksen (2013). Effects of environmental factors on edible oil quality of organically grown Camelina sativa. J. Agri. Food. Chem. 61(13): 3179-3185.
- Koca Y. and İ. Turgut (2012). The effect of different sowing date for grain yield, dry matter accumulation, leaf area index and some growth parameters in maize (Zea mays). J. Adnan. Men. Uni. Agri. Fac. 9(1):1-10.
- Koutroubas A.D., S. Fotiadis and C.A. Damalas (2012). Biomass and nitrogen accumulation and translocation in spelt (Triticum spelta) grown in a Mediterranean area. Field. Crops. Res. 127:1-8.
- Kurasiak-Popowskaa, D., A. Tomkowiaka, M. Człopińskab, J. Bocianowskic, D. Weigta and J. Nawracałaa, (2018). Analysis of yield and genetic similarity of Polish and Ukrainian Camelina sativa Ind Crops. Prod. 123: 667–675.
- Kurt O. (2015): Bitki Islahı [Plant Breeding]. Ondokuz Mayıs University, Faculty of Agriculture in Press, Samsun (Turkey), 212 p.
- Kurt O. and F. Seyis (2008). An alternative oilseed crop: camelina [Camelina sativa (L.) Crantz]. An. J Agri. Sci. 23(2): 116-120.
- Liu Y.C., A.J. Savas and C.T. Avedisian (2013). The spherically symmetric droplet burning characteristics of jet and biofuels derived from camelina and tallow. Fuel. 108: 824-832.
- Lutts S., M. Almansouri and J.M. Kinet (2004). Salinity and water stress have contrasting effects on the relationship between growth and cell viability during and after stress exposure in durum wheat callus. Plant. Sci. 167: 9-18.
- Martinelli T. and I. Galasso (2011). Phenological growth stages of Camelina sativa according to the Extended BBCH Scale. Annals. App. Bio. 158: 87–94.
- Neupane D., J.K.Q. Solomon, E. Mclennon, J. Davison and T. Lawry (2019). Sowing date and sowing method influence on camelina cultivars grain yield, oil concentration and biodiesel production. Food. Energy. Sec.8: e00166.
- O’Neal M. E., D.A. Landis and R. Isaacs (2002). An inexpensive, accurate method for measuring leaf area and defoliation through digital image analysis. J Econ Entomol., 95:1190–1194.
- Peiretti P.G. and G. Meineri (2007). Fatty acids, chemical composition and organic matter digestibility of seeds and vegetative parts of false flax (Camelina sativa) after different lengths of growth. Anim. Feed. Sci. 133: 341–350.
- Poorter H. and A. van der Werf (1998). Physiological Mechanisms and Ecological Consequences, Inherent Variation in Plant Growth. In: Lambers H, Poorter H, van Vuuren MMI, editors. Is inherent variation in RGR determined by LAR at low irradiance and by NAR at high irradiance? A review of herbaceous species. Leiden, (the Netherlands), 309–336 p.
- Poorter H. and C. Remkes (1990). Leaf area ratio and net assimilation rate of 24 species differing in relative growth rate. Oecologia. 83: 553–559.
- Russo V.M., B. D. Bruton and E. Sams (2010). Classification of temperature response in germination of Brassicas. Ind. Crops. Prod. 31(1):48-51.
- Sawyer K. (2008). Is there room for camelina?. Biodiesel Mag. 5(7): 83–87.
- Shipley B. (2002). Trade‐offs between net assimilation rate and specific leaf area in determining relative growth rate: the relationship with daily irradiance. Funct Ecol. 16: 682–689.
- Sintim H.Y., V. D. Zheljazkovg, A. K. Obour, Y. Garcia, A. Garcia and T.K. Foulke (2016). Evaluating agronomic responses of camelina to seeding date under rain-fed conditions. Agro. J. 08: 349–357.
- Srivastava B.K. and R.P. Sing (1980). Morpho-physiological response of garden pea (Pisum sativum) to sowing dates II Growth and development pattern. Indian. J. Horti. 1: 382-389.
- Tagliapietra E.L., N. A. Streck, T.S.M. Rocha, G.L. Richter, M.R. Silva, J. C. Cera, J.V.C. Guedes and A.J. Zanon (2018). Optimum leaf area index to reach soybean yield potential in subtropical environment. Agro. J. 110(3):932-938.
- Taiz L., E. Zeiger, I.M. Moller, and A. Murphy (2014). Plant Physiology and Development 6th Sinauer Associates. California (USA).
- Uzun S. and Y. Demir (1996). Effect of temperature and light on plant growth, development and yield. J. Agri. Sci. 11(3): 201-212.
- Veneklaas E.J. and L. Poorter (1998). Physiological Mechanisms and Ecological Consequences, Inherent Variation in Plant Growth. In: Lambers H, Poorter H, van Vuuren MMI, editors. Growth and carbon partitioning of tropical tree seedlings in contrasting light environmental. Leiden (the Netherlands), 337-361 p.
- Waraich E.A., Z. Ahmed, R. Ahmad, Saifullah, M. Shahbaz and Ehsanullah (2017a). Modulation in growth, development, and yield of Camelina sativa by nitrogen application under water stress conditions. J Plant Nutri. 40(5): 726-735.
- Waraich E.A., Z. Ahmed, R. Ahmad and R.N. Shabbir (2017b). Modulating the phenology and yield of Camelina sativa by varying sowing dates under water deficit stress conditions. Soil. Envir. 36(1): 84-92.
- Yasari E. and A.M. Patwardhan (2006). Physiological analysis of the growth and development of canola (Brassica napus) under different chemical fertilizers application. Asian. J. Plant. Sci. 5(5):745-752.
- Zubr J. (1997). Oil-seed crop: Camelina sativa. Ind. Crops. Prod. 6(2): 113–119.
|